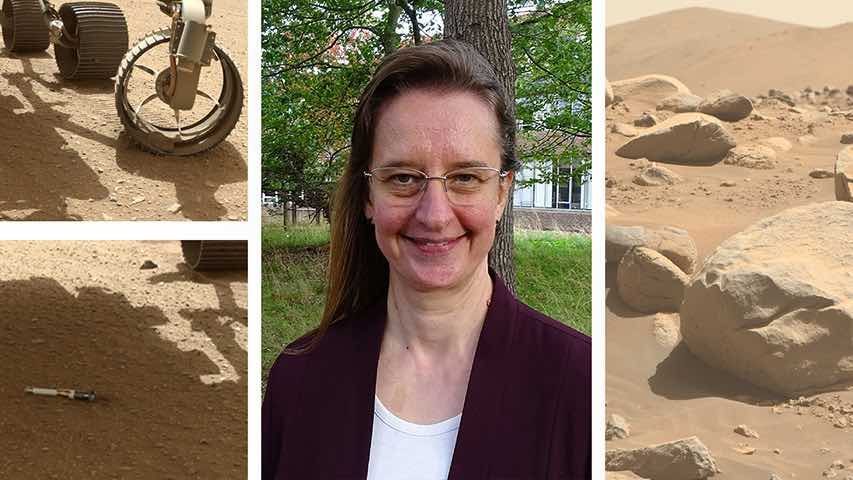
We met Susanne Schwenzer, mineralogist, planetary scientist and Senior Lecturer at The Open University in Milton Keynes (United Kingdom). She is specializes in fluid-rock interactions, and currently focusing on the exploration of Mars as a member of the NASA’s Curiosity Mars Rover team. Schwenzer is also one of the six Europeans of the NASA/ESA’s Mars Sample Return Science Group.
Mars Sample Return is the most important and difficult robotic mission of the history of space exploration: the goal is to go and get the Martian samples, that NASA’s Perseverance Mars Rover is currently collecting and depositing on the surface of Mars. The plan is to bring them to Earth by 2033. The Perseverance rover, which landed on Mars in 2021, is located in Jezero crater, precisely in the ancient Mars river delta, where 3 billions of years ago a river of water flowed out. So, scientists need to figure out whether there was life on Mars in the past, or whether there is still microbial life today.
How was your passion for the study of water through the terrestrial and Martian rocks born?
I am a mineralogist by training and studied water-rock reactions here on Earth for my Diplom (Masters) thesis at University of Mainz, Germany. I then went on to do a PhD on a different set of volatiles and a different planet: noble gases in Martian meteorites. But some of those noble gas ‘finger prints’ are likely to record water-rock reactions too. Combining the two, mineralogy of water-rock reactions and Mars, I found my new passion in studying those reactions on Mars. But of course, Earth still plays a vital role, too, as we can study many of the processes here on Earth on rocks similar to the ones on Mars and then learn about both planets in the process.
When you study the terrestrial and Martian rocks, how do you understand if there was water in the past?
Mineralogy is key to understanding what happened to a rock. If you go to Mt Etna and pick up a piece of the new rock that is from the lava that just cooled, you will see it is black and shiny, and it has some vesicles – open and empty little bubbles that ‘froze’ in place when the lava cooled and formed this rock. They were originally filled with gas from the magma, now they are filled with air. If you go to a place on the same volcano and look at rocks where vegetation has already started to grow on, you will see that the rock looks dull, and you may even find some of the vesicles filled with white material. Those are newly formed crystals from the reaction of the rock with the water from the rain, but also other chemicals, for example the CO2 dissolved within it from the atmosphere or the organic acids that come from the plants and microbes in the environment.
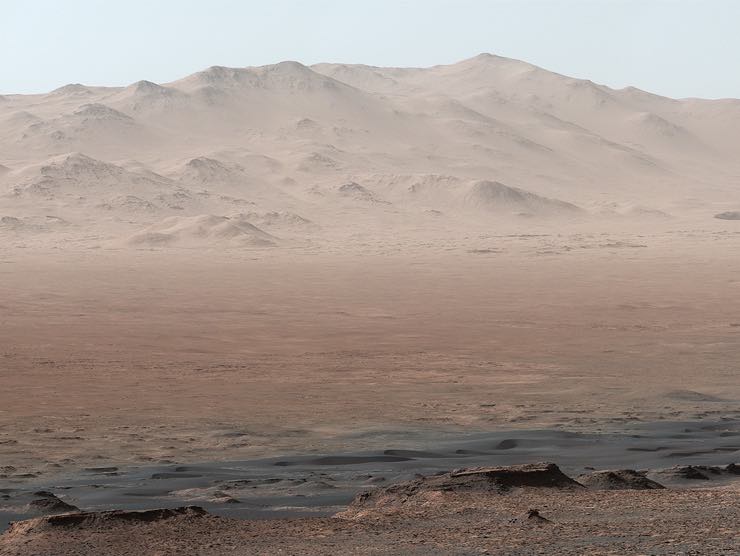
Credits: NASA/JPL-Caltech/MSSS
This is just one example to what happens when water and rock react. But it is one that everyone can study when hiking around Mt Etna (when it is safe to do so, of course!). When I look at a rock, I often do not have the luxury to have the fresh and the altered version to compare with, I am just presented with the altered version and have to piece together what happened. That’s a little bit like a crime scene: we are presented with the result of the action of water but that water is long gone, most of the time millions of years, but sometimes even billions of years!
The key is to first to what a detective would do, too: observe, collect all the evidence. A mineralogist’s tools are first of all their eyes, looking at the rock, is it shiny? How many different colours and shapes do I see? Next we look with a hand lens and then a microscope. That already allows us to understand the minerals in the rock, and use our knowledge of which minerals form from lava or other geologic processes as primary minerals, and which ones are typically formed by water-rock reactions, the so called secondary minerals. It gives a good overview. We then can refine that overview by using analytical instruments, and that will give us very deep insights into the chemistry of each of the minerals. We might also find some minerals that we could not see at the resolution of a microscope, because they are even smaller.
Once we understand the chemistry of all the minerals and can divide them into the two groups, those that are primary and those that are secondary, we understand the reactions that have happened. But there is one actor we cannot observe: the water! It’s long gone (just as the criminal at the crime scene, right?). We then use a tool called thermochemical modelling to find out what the conditions of this water must have been to form the secondary minerals we observe from the primary ones that once were there. This allows us to find out about the temperature this happened at, but also about the water itself: was it salty or not?
When there are the fluid-rock interactions, what does it happen to the rock?
When a fluid reacts with a rock, it dissolves the minerals within that rock. The fluids are usually water with some dissolved chemicals in it: CO2 from the atmosphere, maybe some sulphuric acid, too, if there is a volcano underneath. If you think of the Campi Flegrei and the smell around them, you have a good image of the gases that can come from a volcano and can become dissolved in water – groundwater or rainwater alike. Depending on the chemistry and temperature, the fluid will dissolve just a little or a lot of the minerals of the rock.
As it does so, more chemical elements are entering the fluid. At some point the concentration of some of these elements and chemical compounds will become so high that new minerals start to form. The first two elements to form new minerals are usually iron and aluminum. You might have found rocks with reddish or yellow-reddish surface stains: those are iron oxides and iron hydroxides, which are one of the first minerals to form when rock dissolved in water.
The while crystals I mentioned earlier are often zeolithes, they are formed from the silica and aluminum in the rock, but also have some other elements such as sodium and potassium in them. Which new minerals form is highly dependent on two things: the composition of the rock, for example whether it was a basalt from Stromboli or a granite from Sardinia, and the nature of the water, for example, how acidic or basic it was and what its temperature was. If it was a basalt, elements such as iron and magnesium will be more concentrated in the solution and iron oxides will likely form as a result of the water-rock reactions. A granite has very little iron but a lot more calcium than a basalt, making it more likely to form a calcium carbonate as secondary mineral. So, the answer what actually happens is very complex and often takes weeks to figure out!
The scientists can be something of special because you can understand if there was water on Mars 3 billion years ago, studying only a little rock!
Mineralogy, just like biology, too!, is a science based on very careful observations. If we have a rock in front of us, we can find out about many details just from looking, often with the help of microscopes, and finding out the minerals that are present, but also their relationships – which one was there first, what happened to it. One can see when a mineral was dissolved at it edges, and what new minerals might have formed in which order.
Now you are also a science team member of the NASA’s Curiosity Mars Rover. What are the most important discoveries that you did during the Curiosity mission?
A mission is a team effort, nothing is done by any single person. I feel incredibly privileged being part of a large team of scientists and engineers who together explore Gale Crater on Mars. The Curiosity rover has made some incredible discoveries over the years, and it continues to do so! (Here are some summaries by the team: Curiosity Celebrates 10 Years on Mars | NASA). If I should pick my two personal favorites then it is the water rock reactions, of course.
My first mention is the publication I led on how the calcium sulfate veins Curiosity found early in the mission formed through water-rock reactions followed by precipitation, re-dissolution and precipitation in the veins (Fluids during diagenesis and sulfate vein formation in sediments at Gale crater, Mars – Schwenzer – 2016 – Meteoritics & Planetary Science – Wiley Online Library). The second mention is the application of noble gases, more specifically K-Ar dating to the rocks on Mars and the water rock reactions that happened to them: we found out that the main minerals in the sediment formed about 4 billion years ago, but jarosite – a secondary alteration mineral – might have formed as late as 2.6 billion years ago.
The study was led by my team-colleague Peter Martin, who did all the calculations to combine APXS measurements of potassium with Ar measurements from the SAM instrument (A Two‐Step K‐Ar Experiment on Mars: Dating the Diagenetic Formation of Jarosite from Amazonian Groundwaters – Martin – 2017 – Journal of Geophysical Research: Planets – Wiley Online Library). But there are also many discoveries made by the team, where I am not involved in the science, but where I am really excited about what they find. Have you seen the clouds on Mars? (NASA’s Curiosity Rover Captures Shining Clouds on Mars). I am just so happy and excited to be part of the team, and two or three times a month help put together the plans that will be sent to the rover to perform all the measurements on Mars – it’s what we colloquially call ‘drive a rover on Mars’, which is done by a team of engineers and scientists each planning day.
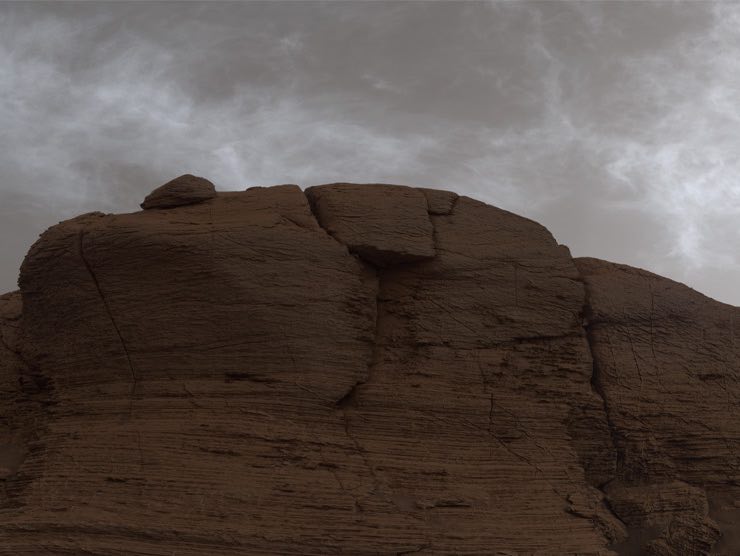
Credits: NASA/JPL-Caltech/MSSS
What emotions do you feel when you personally study the planet Earth (like for example Utah desert or the Atacama Desert), with the objective to understand another planet as Mars? What is the secret to work with passion of all the entire life?
When I work on Earth or on Mars to study the rocks, in the field and in the laboratory, I am driven by curiosity. I just want to find out more about what is in front of me. But desert landscapes are also fascinating in their own right, the emptiness, the hostility to life – yet, in every desert you will find life that has made those hostile places their home. When I was in the Atacama, at very high altitude, with no water to be seen, walking around doing geology, I stumbled upon an entrance to a little burrow. Of course, I didn’t dig to see who lived there, but I saw the fresh marks of entrance, probably of a little rodent. Isn’t that amazing?
You are one of the six Europeans of the Mars Sample Return science group. What is the difference between the Martian meteorites fallen on Earth and the mars samples who is gathering NASA’s Perseverance Mars Rover? Why is it so important to bring mars samples to Earth?
This is a great question, because we have a collection of meteorites here on Earth, which are known to be from Mars. We study them on a daily basis and we have found out a lot about Mars already through studying those meteorites. And we will continue to study them, even after the returned samples have arrived here on Earth! They are very valuable messengers sent to us from Mars for free! But that also is their problem! To get away from Mars, they need to be part of a meteorite impact on Mars. There are lots of large craters on Mars, when you look at images of the Martian surface. The biggest ones will have caused material to launch from the Martian surface into space. And that process ‘filters’ for the rocks that can survive those violent processes and those that would be destroyed and become small dust that never makes it to Earth.
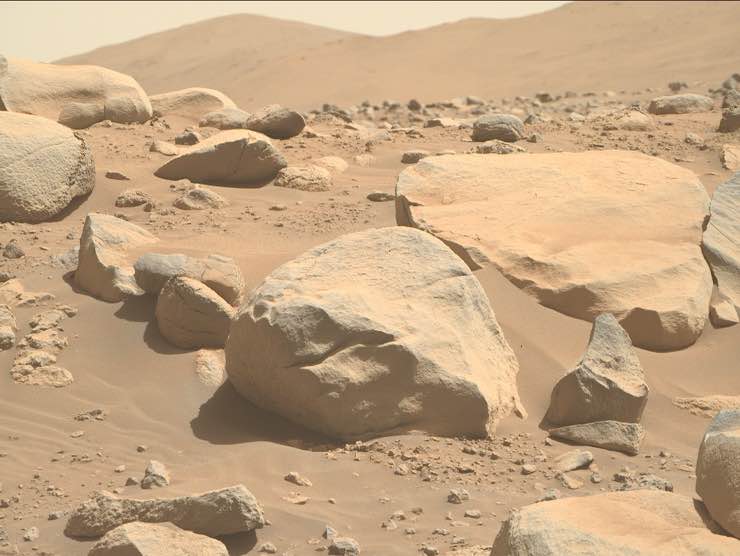
Credits: NASA/JPL-Caltech/ASU
So, we have a selection, and in fact we do not have any sedimentary material in the meteorite collection! But – as we know from the rovers – there are many different types of sediments on Mars! So, we are missing some important parts of our collection and that is one aspect that Mars Sample Return will change. But there is more! When you think of that rock that was launched from Mars then you have to next think of it’s journey: It does not take a direct trajectory, but instead travels around the sun for some while until it by chance meets the Earth on its orbit and falls onto Earth.
That long duration in space means it has been without any protection from the radiation of space for a very long time. That destroys organic materials – or at least alters them. And it also alters some other signatures in the rock and obscures what we could be learning from Mars. After the meteorite arrives on Earth, only very few are found within hours or days of their fall. Most of them spend decades or more in the hot and cold deserts of Earth and are subject to alteration – water rock reactions – here on Earth, which also obscures some of the signatures that would otherwise allow us to learn about Mars. But that’s not even all of the problems!
Probably the biggest one – apart from the missing sediments – is that we do not know where the meteorites come from! For a geologist, to piece together what we can learn from that rock in hand with the wider geologic history of the planet, that location information is vital! So, we have very valuable samples in hand already, and they will continue to be treasured and investigated, of course. But what sample return will add is a set of carefully collected samples where we know the exact context of their location and that also include many materials that we do not have in the meteorites.
If you look at the cache that Perseverance laid down at Three Forks in Jezero Crater (Perseverance’s Three Forks Sample Depot Map (nasa.gov); Perseverance’s Three Forks Sample Depot Selfie – NASA Mars Exploration; NASA’s Perseverance Rover Shows Off Collection of Mars Samples; Mars Rock Samples – NASA Mars Exploration) you will see that the Rubion sample is an atmospheric sample, Crosswind Lake is a sample of loose material called regolith, Malay, Coulettes, Montdenier and Atsá are igneous samples and Mageik, Bearwallow and Skyland are sedimentary samples.
Each of those samples has been taken after carefully mapping the area from orbit and investigating it with all instruments at the rover’s disposal. Perseverance has a twin-set of samples on board and will continue to add to the collection. When those samples arrive on Earth, we will have a carefully documented set of samples of a wide variety of rocks and other materials, such as the atmosphere, here on Earth to study with all the instrumentation that we have available to us here, and they will only have been exposed to space for a few month, not hundreds of millions of years!
- Cover image: Susanne P. Schwenzer (central photo: lpi.usra.edu); side photo: NASA/JPL-Caltech/ASU/MSSS (first image) / NASA/JPL-Caltech/ASU/MSSS (second image)