We met Abraham (Avi) Loeb, “Frank B. Baird Jr. Professor of Science” at Harvard University in Cambridge, Massachusetts (USA). In December 2012, Time magazine selected Loeb as one of the 25 most influential people in Space. In June 2020, Loeb was sworn in as a member of the President’s Council of Advisors on Science and Technology (PCAST) at the White House.
Abraham (Avi) Loeb and Sunny Vagnozzi recently published an article in The Astrophysical Journal Letters (to read it click HERE), in which they explain how the theory of cosmic inflation could be rejected, thanks to the existence of a signal in the Cosmos, called Cosmic Graviton Background (CGB). The challenge is to find this signal, the CGB. If they can find it, we will have proof that the origin of the universe did not happen as we think today. In fact, the Big Bang theory does not allow the existence of the CGB, so its detection would exclude the Big Bang and cosmic inflation.
What is the most important riddle of cosmic inflation? Why don’t many scientists all agree on cosmic inflation?
The initial version of inflation developed in the early 1980s explained the flat geometry, the near uniformity and isotropy of the Universe and the origin of structure in it as a result of quantum fluctuations. It made testable predictions and was falsifiable. But as the theory was explored further, it was realized that it can accommodate all possible outcomes. First, one can always reverse engineer an inflationary model given an observed outcome. Cosmologists came with variants of the model that can accommodate a non-flat universe. Second, it was realized that if one starts from random initial conditions of large inhomogeneities, inflation may not be possible. Third, quantum fluctuations can lead to any outcome. In the words of one of inflation’s pioneers, Alan Guth: “Anything that can happen will happen an infinite number of times”. Given this statement the theory is not falsifiable because we have no way of calibrating the likelihood of different outcomes and therefore no way of ruling out the theory based on the observed properties of our universe.
String theory added the notion of a huge number of possible vacuum states, suggesting that our cosmological parameters were selected such that we would exist, the so-called the anthropic argument. To many scientists, this is not a satisfactory explanation because it cannot be proven wrong by a hypothetical data set.
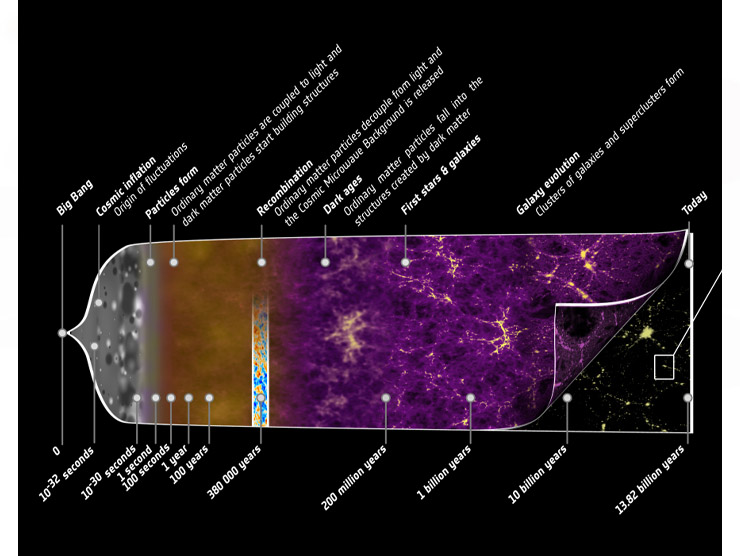
Credit: NASA
To rule out the “cosmic inflation theory” we need to find the “cosmic graviton background (CGB)”. How will you find the CGB?
A challenging approach is by measuring precisely the amount of radiation in the Universe based on the cosmic expansion rate, as measured by fluctuations in the cosmic microwave background. The graviton background add a little bit to the cosmic microwave and neutrino backgrounds, both relic from the early Universe. A more promising approach is by converting gravitons to photons in a strong magnetic field. Below are some general comments about the various radiation background in the Universe.
The cosmic microwave background (CMB), relic from the Big Bang, accounts for a percent of the static noise visible as “snow” in old-fashioned television sets. The photosphere that last-scattered this radiation 400,000 years after the Big Bang, had a temperature of 3000 degrees Kelvin, half of the Sun’s effective temperature. The spherical last-scattering surface around us marks the boundary of the transparent volume of the observable Universe. We cannot see farther. Gladly, the first stars formed about a hundred million years later. The Webb telescope will search for them through its longest exposures.
The actual edge of the observable Universe is at the distance that any signal could have travelled at the speed-of-light limit over the 13.8 billion years that elapsed since the Big Bang. As a result of the expansion of the Universe, this edge is currently located 46.5 billion light years away. The spherical volume within this boundary is like an archaeological dig centered on us: the deeper we probe into it, the earlier is the layer of cosmic history that we uncover, all the way back to the Big Bang which represents our ultimate horizon. What lies beyond the horizon is unknown. Within the thin spherical shell between the Big Bang and the microwave background photosphere, the Universe was opaque to light. Nevertheless, we can probe into this layer. Neutrinos have a weak cross-section for interactions, and so the Universe was transparent to them back to approximately a second after the Big Bang, when the temperature was ten billion degrees. The present-day Universe must be filled with relic neutrinos from that time.
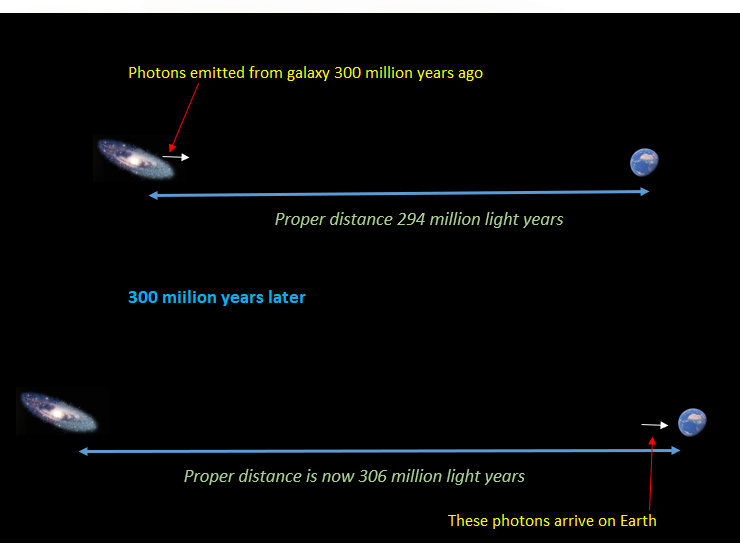
Credit: https://explainingscience.org/
The expansion of the Universe cooled the neutrino background to a present-time temperature of 1.95 degrees above absolute zero, comparable to the 2.73 degrees of the cosmic microwave background.
Can we probe even deeper into our cosmic archaeological dig? In principle, yes. Gravitational radiation has weaker interaction than neutrinos. So much so, that the Universe was transparent to gravitons all the way back to the earliest instant traced by known physics, the Planck time: 10 to the power of -43 seconds, when the temperature was the highest conceivable: 10 to the power of 32 degrees. A proper understanding of the Planck epoch requires a predictive theory of quantum gravity, which we do not possess.
If gravitational radiation was thermalized at the Planck time, should there be a relic background of thermal gravitational radiation with a temperature of about one degree above absolute zero?
Not so, according to the popular theory of cosmic inflation, which suggests that the Universe went through a subsequent phase of exponential expansion that diluted all earlier relics to undetectable levels. Inflation was theorized to explain various fine-tuning challenges of the Big Bang model. It also explains the origin of structure in our Universe as a result of quantum fluctuations. However, the large flexibility displayed by numerous possible inflationary models raises concerns that the inflationary paradigm as a whole is not falsifiable, even if individual models of it can be ruled out. Is it possible in principle to test the entire inflationary paradigm in a model-independent way?
Credit: Explaining Science (YouTube channel)
In our new paper with Sunny Vagnozzi, we showed that future detectors could potentially discover the one-degree gravitational wave background, if it exists. This cosmic graviton background adds to the cosmic radiation budget, which otherwise includes microwave and neutrino backgrounds. It therefore affects the cosmic expansion rate of the early Universe at a level that might be detectable by the next generation of cosmological probes.
A discovery of the thermal graviton background holds the potential of ruling out the inflationary paradigm and bringing us back to the drawing board of how the Universe began. Given that the interiors of black holes are hidden from view and are risky to venture into, the early Universe might represent our best opportunity for testing predictive theories of quantum gravity.
There is no reason to assume that our cosmic roots started at the Planck time. Albert Einstein was inclined to think that our past timeline should have no beginning. But to his dismay, he later realized that the equations of the General Theory of Relativity do not admit a stable static solution, and moreover — the actual Universe appears to be expanding. His philosophical preference for no beginning might be validated by the ultimate theory to combine General Relativity with Quantum Mechanics, which could explain what predated the Big Bang.
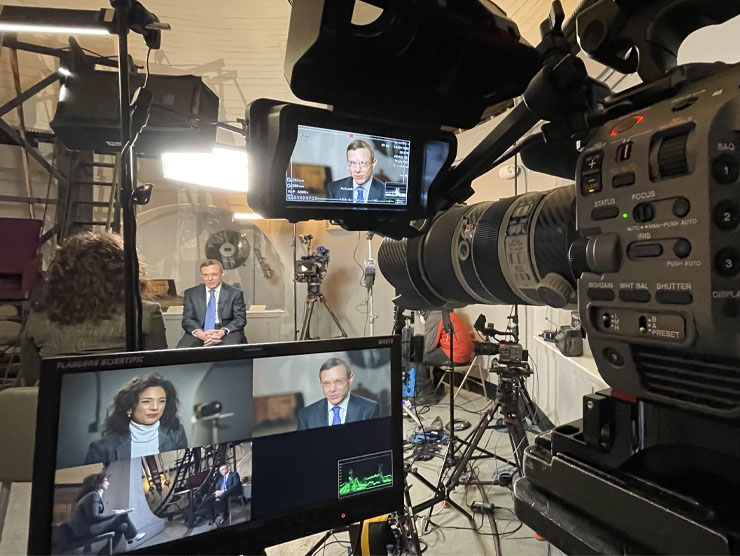
Credit: personal collection (Rob Massey/Pulse Media; 8 febbraio 2021)
A predictive theory of quantum gravity could rid us of the Big Bang singularity. But just as with the development of quantum mechanics, we might need guidance from experimental data or else we will have too many possible theoretical scenarios.
Here’s hoping that the new paper I wrote with Sunny will lead to progress by eliminating theoretical possibilities. Scientific knowledge encapsulates what actually exists in the cosmos out of the many possibilities that could have existed. Sometimes, the most beautiful possibilities are ruled-out, even if they were conceived by the most brilliant scientists on planet Earth, like Albert Einstein. As known from social media or politics, beauty and truth are not necessarily the same.
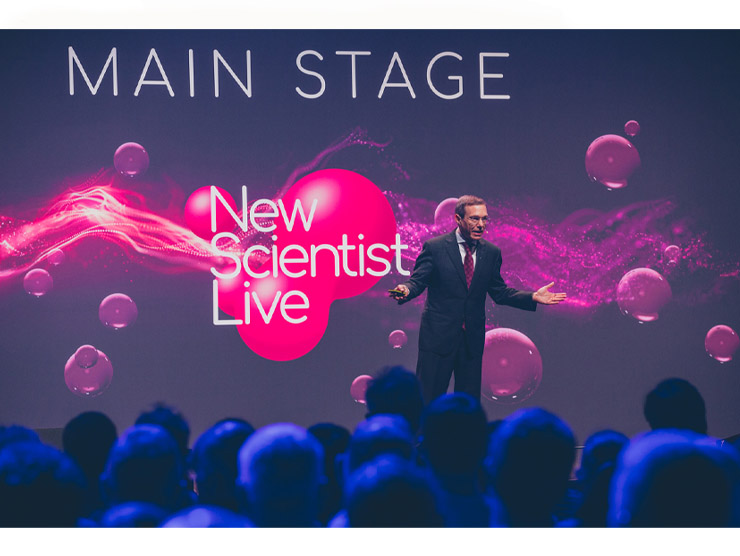
Credit: Loeb’s photo collection (October 12, 2019)
READ ALSO –> James Webb Telescope experts answer our questions
If I could find the CGB it would change the origin of the Universe. And if the Universe was not born from the Big Bang, how to explain dark energy, which accelerated the expansion of the Universe?
The dark energy represents the ground state of the vacuum in the present-day Universe. We might be able to explain it once we will have a predictive theory of quantum gravity.
During your prestigious career, you have also served as a member of the President’s Council of Advisors on Science and Technology (PCAST) at the White House, you are the founding director of Harvard’s Black Hole Initiative and Time magazine has listed you among the 25 most influential in space. What is the greatest emotion you remember from your career?
It was the surprise that the first three interstellar objects that were discovered over the past decade, two of which were meter-size meteors that I discovered with my student, appear to be anomalous. They do not shar the properties of familiar asteroids or comets in the solar system. Just as in the case of dark matter and dark energy, the Universe is telling us that what exists outside the solar system is different from what we find in our backyard.
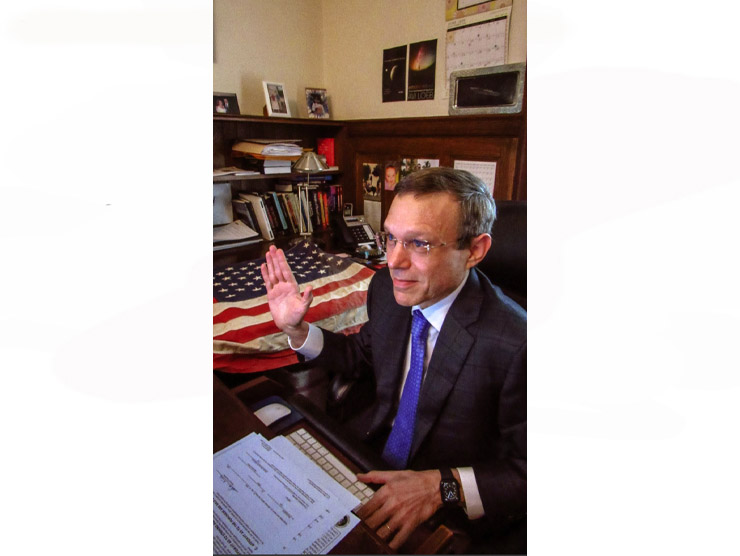
Credit: Lotem Loeb (June 30, 2020)
READ ALSO –> Roman Space Telescope, two NASA astrophysicists answer our questions
Your work also focuses on extraterrestrial life. What are the most important anomalies of the famous interstellar object Oumuamua? And what do you think about possible extraterrestrial life?
We now know that a significant fraction of all Sun-like stars have a planet the size of the Earth roughly at the Earth-Sun separation. Moreover, most Sun-like stars formed 10 billion years ago, whereas the Sun formed only 4.6 billion years ago. This implies that the dice of intelligence was roled billions of times in the Milky Way galaxy alone. It is very likely that Albert Einstein was not the smartest scientist who ever lived in the past 13.8 billion years since the big Bang. Similarly, Elon Musk was not the greatest rocket builder over the Milky Way history. Since it takes less than a billion years for a chemical rocket of the type that we launched, to traverse the Milky Way, we should be able to find through our telescopes interstellar probes from advanced civilizations that predated us. This is the goal of the Galileo Project that I am leading at Harvard University. We are starting to get data from our new suite of telescopes monitoring the sky.
Credits: NASA/JPL-Caltech
We also plan to retrieve the fragments of the first interstellar meteor near Papua New Guinea by summer 2023. We know that this meteor was tougher than iron from the low elevation where it disintegrated. We would like to find out whether it was made of an artificial alloy like stainless steel. I promised the curator of the Museum of Modern Art in New York City, Paula Antonelli, that if we find a technological gadget from another civilization, I will bring it for display. It would represent modernity for us even though it may represent ancient history for the senders.
- Cover image credit: Photo in the center: Washington Post. Credit: Adam Glanzman (Posted: March 27, 2019); Photo in the left: TAKE 27 LTD/SPL; Photo in the right: Goddard Space FLight Center/Flickr CC BY 2.0